Cell and gene therapy (CGT) products represent a frontier in modern medicine, offering precision treatments. Yet their development is affected by multifaceted challenges including chemistry, manufacturing, and controls (CMC). This article provides a comprehensive exploration of the complexities involved in CMC development and considers its role in establishing quality, safety and efficacy.
We have endeavoured to explore the key elements of CMC pertaining to cell sourcing, vector development, manufacturing and control of drug products. Furthermore, we have delved into other critical factors such as raw materials/reagents selection, manufacturing process changes and comparability studies. This article also highlights the need for sponsors to periodically revisit the product development strategy to be in line with clinical development.
Regulatory Rapporteur
January 2024 | Volume 21 | No.1
Licence notice
Copyright © 2015-2023 The Organisation for Professionals in Regulatory Affairs Ltd. T/A Regulatory Rapporteur − All Rights Reserved. This work is licensed to Allison Gillespie for the purposes of academic and personal reasonable use only.
Notwithstanding this licence, no part of materials published in Regulatory Rapporteur may be reproduced without the express written permission of the publisher.
As a general rule, permission should be sought from the rights holder to reproduce any substantial part of a copyrighted work. This includes any text, illustrations, charts, tables, photographs, or other material from previously published sources.
To obtain permission(s) to re-use content published in Regulatory Rapporteur please email publications@topra.org.
To join TOPRA please click here.
Introduction
CGT products can transform healthcare by treating oncology, inherited diseases and chronic conditions. The exponential development of gene therapies offers a potential one-time, lifelong cure. CGT products are often discussed interchangeably but there are major differences. Cell therapy refers to the transfer of autologous or allogeneic cellular material into a patient for medical purposes. The cellular material can be administered topically or as injectables, infusions, bioscaffolds or scaffold-free systems.[1]
Cell therapy primarily centres on immune and stem cells, with a major focus on CAR-T and natural killer (NK) therapies. The immune cell pipelines have expanded to include gamma delta (γδ) T-cell therapy, CAR-Treg therapy, CAR-M therapy, TCR-based therapy and T-cell antigen coupler (TAC) therapy.[2] On the other hand, in gene therapy, the genetic material (i.e. DNA or RNA) is administered via a carrier, known as a vector.[3]
CMC plays a key role throughout the development of CGT products and sponsors are required to provide CMC information describing product manufacturing and testing to assure safety, identity, quality, purity and strength, including potency of the product.[4]
Overview of approved CGT products
As of October 2023, 65 non-genetically modified cell therapies and 53 gene therapies, including 26 RNA therapies, were globally approved for clinical use.[5] The first human gene therapy drug for an inherited retinal dystrophy, was approved by the US FDA in 2017.[6] A diverse range of cell- and gene-based therapies are currently available in the market. These therapies extend their reach to address life-threatening conditions pertaining to oncology, osteoarthritis, advanced melanoma, ophthalmic issues, immunodeficiencies, cardiovascular ailments, neurological disorders and genetic disorders. In the last two decades, several advanced CGTs designed to target rare genetic diseases have demonstrated long‐lasting efficacy for treatment of severe and previously incurable diseases and returning patients to a normal life.
As of October 2023, 65 non-genetically modified cell therapies and 53 gene therapies, including 26 RNA therapies, were globally approved for clinical use…
In the US and the EU, some CGT products were withdrawn for clinical or commercial reasons. This includes the withdrawal of efficacious and approved products, due to unsatisfactory reimbursement negotiations with individual European states. Several sponsors had to drop approved advanced therapy medicinal products (ATMPs) post-approval and reimbursement negotiations, especially for rare diseases with very few patients. There are currently 3,866 therapies in development from non-clinical through pre-registration; 53% of those are gene therapies, 25% RNA therapies, and 22% non-genetically modified cell therapies.[5]
The regulatory landscape
In the EU, CGT products fall under ATMPs, comprising gene therapy, somatic cell, tissue engineered and combined ATMPs. Regulation (EC) No 1394/2007 and Directive 2001/83/EC provide detailed classifications, shaping the regulatory framework. A joint action plan in October 2017 streamlines procedures, while the EMA’s Committee for Advanced Therapies (CAT) assesses and guides ATMP development. New regulatory pathways, such as the priority medicines scheme (PRIME), along with new meeting formats, have been established to expedite the development and review of submissions. All ATMPs undergo centralised evaluation for EU-wide authorisation (Regulation (EC) 726/2004).
In the US, the Center for Biologics Evaluation and Research (CBER) oversees CGT products. The 21 Century Cures Act, enacted in 2016, introduced the Regenerative Medicine Advanced Therapy (RMAT) programme. RMAT covers drugs involving cell therapy, tissue engineering or combination products which address serious or life-threatening diseases.
Other countries including Australia, Brazil, Canada, China, India, Israel, Japan, Mexico, Republic of Korea, Saudi Arabia, Singapore and Switzerland have also recently introduced regulatory frameworks for CGT. However, global alignment of regulatory pathways is crucial for the development and accessibility of these therapies.[7]
CMC development and challenges
Raw materials/reagents
It is recommended to use the highest quality raw materials and reagents available at all stages of product manufacture. When sourcing reagents, sponsors shall have established procedures confirming that each reagent is of suitable quality for use in manufacturing process. Examples of reagents include foetal bovine serum, trypsin, digestion enzymes (e.g., collagenase, DNase) growth factors, cytokines, monoclonal antibodies, antibiotics, cell separation devices and media components. These reagents can affect the safety, potency and purity of the final product, especially by introducing adventitious agents.
Sponsors shall provide information verifying the source, safety and performance of the reagent and establish a qualification programme that includes safety testing (sterility, endotoxin, mycoplasma, and adventitious agents), functional analysis, purity testing and assays (e.g. residual solvent testing) to demonstrate absence of potentially harmful substances. The extent of testing will depend on how and when the specific reagent is used in the manufacturing process.
Cell sourcing
Cell sourcing is a crucial aspect of cell therapy product development and involves obtaining cells for therapeutic use. The choice of cell source significantly impacts the safety, efficacy and scalability of the therapy. Cells are sourced from the affected individuals (autologous) or from healthy donors (allogenic) depending on the type of therapy. The cell selection is based on phenotype, desired cell population, genetic modifications, in vitro cell expansion and subsequent patient infusion. The cell therapy development process is outlined in Figure 1.
Note: Process similar for other genetically-modified and non-genetically modified cell therapies, including but not limited to: stem cells, CAR NK and tumour infiltrating lymphocytes (TIL).
Autologous therapies
Autologous therapies using a patient’s own cells reduce the risk of immune rejection and are commonly used in treatments like CAR-T cell therapy.[8] In such product development stages, starting materials availability is limited as each batch is unique. So, the sponsor should be cautious from cell isolation to re-infusion. Cell culturing/expansion is a critical step, and the sponsor should prioritise achieving acceptable population doubling ranges and monitoring cell concentration and viability throughout the process.
After batch manufacturing, the product is shipped to the patient site and sponsors should establish validated transportation conditions as per the product nature. These therapies have a short ex vivo half-life, presenting a significant challenge. To address this, manufacturing facilities, involving genetic modification, expansion and cryopreservation shall be located close to clinics for efficient logistics. Autologous therapies require two clinical steps (cell isolation and re-infusion) making manufacturing time and logistics critical.
Allogenic therapies
Cells sourced from donors can be further harvested, sorted, genetically modified and stored under appropriate conditions. Once harvested, the required cells are selected to produce multiple batches from the same donor, and this is particularly useful for patients requiring second or multiple treatments. The challenge with allogenic cell therapy is that it requires matching for compatibility and potential immunosuppression to prevent rejection.[8] Allogenic therapies have similar CMC challenges as autogenic therapies. Additionally, allogenic therapies include the need for sponsors to prioritise donor screening and processing in accordance with established regulatory guidelines and more traceability is required on manufactured batches from donor to patient, if more than one dose is recommended.
Viral and non-viral vectors
Viral and non-viral vectors play key role in gene therapy, each offering unique advantages. Viral vectors, such as lentiviruses and adenoviruses, are valued for their exceptional transduction efficiency, enabling effective gene delivery.[9] These are proven in penetrating the cell nucleus and delivering genetic materials. On the other hand, non-viral vectors are often considered safer as they avoid potential immune responses or genomic integration risks associated with viral vectors.[10] They are easier and more cost-effective to manufacture, accommodating a wide range of therapeutic genes and potentially presenting simpler regulatory pathways. The choice between viral and non-viral vectors depends on the specific requirements and safety considerations of the gene therapy application at hand.
The selection of suitable and well-characterised cell lines for vector manufacturing while maintaining strict aseptic culture conditions is critical. The helper/packaged cell lines or stable/produce cells were used to avoid issues arising from the use of conventional cell lines. It is challenging to achieve consistent cell expansion, differentiation across different batches. Variability in cell behaviour and response to differentiation cues necessitates meticulous optimisation to ensure reproducibility. Scaling up cell expansion and differentiation from laboratory scale to clinical or commercial scale is complex. Adapting processes for large-scale bioreactors while maintaining cell quality and phenotype remains a challenge.
Host cell transfection
While introducing the genetic materials into host cells, such as plasmids or viral DNA, the sponsor should develop plasmid DNA of good manufacturing practice (GMP) grade to obtain high purity levels and it should be free of process related variants and impurities. There are two main techniques that are used in process transfection: transient and stable transfection. Transient transfection is the conventional process where the transfected DNA is integrated into the genome and remains in nucleus i.e. it is not passed onto the next generation. This vector production is suitable for research and may not be suitable for large scales, whereas stable transfected DNA integrated into the genome is carried stably from generation to generation. This method gives high yield and long-term manufacturing capability.[11]
Cell culture
The host cell transfected with a desired gene is cultured under suitable conditions with due consideration to pH, temperature, dissolved oxygen concentrations and nutrient supply in adherent cell culture or suspension cell culture. To obtain a high yield of viral vector, culture conditions need to be optimised. Adherent cell culture methods can produce enough viral vectors for small batch sizes, which is useful for lab scale manufacture at orphan drug demand levels. These methods may not meet commercial demands for larger numbers of patients.
Challenges could involve utilisation of media containing serum, requirement of scale-out techniques and increased manufacturing expenses. These can be addressed by replacing adherent cell culture with suspension cell culture methods.[12] Process control strategy involves implementation of in-process measures and critical controls that are carefully defined and established throughout the manufacturing process. These controls are pivotal elements designed to monitor and ensure the quality, consistency and integrity of product manufacturing, contributing to the overall efficacy and safety of the final product.
Downstream process
Downstream processing includes cell lysis, clarification/depth filtration, buffer exchange e.g. by tangential flow filtration (TFF) and purification. Cell lysis is performed by using physical or chemical methods to release the viruses. Vector yield can be reduced due to formation of aggregates/precipitation and may impact the safety and or efficacy. Prolonged use of detergents for lysis may cause toxicity and careful selection of lysis methods is important to avoid any impact on vector integrity.
Clarification and depth filtration methods remove cell fragments and debris. Filter selection should match vector sizes to maximise vector yield. Clarified harvest sample conditions shall align with chromatography column equilibration buffers, requiring buffer exchange through TFF. The key challenge in purification steps is to separate the enriched capsids from partially filled and empty capsids because both are similar in size and electrical charge. Sponsor should focus on capsid properties like isoelectric point (pI value) and impurities (empty and partially filled capsids).[13]
Manufacturing processes must be well established and controlled at all stages to ensure the cell therapy products meet the appropriate standards. Many cell therapy products cannot be terminally sterilised and usually rely on aseptic processing. The appropriate controls for aseptic processing in the manufacturing process should be in place to ensure the product is free of microbial contamination at all stages of manufacturing.
Analytical characterisation
Many CMC issues during product development or from regulatory authority reviews revolve around deficiencies in testing and quality issues. This is due to inadequate characterisation and analytical techniques for product purity and potency assays. Selection of appropriate analytical methods is crucial to comprehensively characterise CGT products in terms of assessment of quality, potency, purity, safety and identity. It is important to seek regulatory authority advice to establish appropriate analytical methods for measuring, monitoring and characterising product quality throughout the product development stage. Methods shall be suitable for their intended use and qualified during product development stages. Lot release tests should include validated assays for quality, identity, safety, purity, viability, cell number/dose and potency.
Developing appropriate analytical assays for CGT products presents several significant challenges due to the unique nature of these therapies. Figure 2 demonstrates some of the key challenges.
Sponsors face unique challenges in identification of critical quality attributes (CQAs) and critical process parameters (CPPs) due to manufacturing process complexity and analytical procedures used for the in-process and release testing. Knowledge on CQA and CPPs are also important to qualify the reference standard (RS) which requires frequent testing. The challenge here for cell therapy products is the material limitation as multiple batches cannot be evaluated to choose the best candidate RS because it is possible that there might be only one drug substance /vector to use as RS.
During early product development stages, it is challenging for sponsors to set acceptance criteria with limited manufacturing experience and understanding of product attributes. However, it is recommended to set appropriate acceptance limits, even if they were wider limits, based on the available primary lot data, supporting data (e.g. of earlier lab scale) and product knowledge attained. These need to be revisited during subsequent development stages to narrow down as more information on the product or process is gained.
Analytical methods employed should be qualified and validated based on development stage(s). It is recommended to qualify the methods during clinical phases I and II/first-in-human and perform comprehensive validation before beginning phase III trials and marketing applications. Sponsors encounter challenges at analytical validation stages due to the complexity and inherent variability of CGT products. This shall be addressed by limiting the sources of variability in assays with proper design at the method development phase.
Sponsors face significant challenges in the development of potency assays due to the high complexity of CGT products. Some of the factors which hinder the finalisation of potency assay are:
- the inherent variability in the starting product, due to the autologous and allogenic donor variability, cell heterogenicity and error prone replicating viruses
- limited material for testing
- limited stability
- lack of appropriate reference standards as they can be autologous cellular material or novel gene therapy vectors
- complex mechanism of action
- multiple active ingredients, such as multiple cell lines combined in final product, heterogeneous mixtures of peptide pulsed tumour and/or immune-modulatory cells and multiple vectors used in combination
- the potential for interference of the active substance leading to variability in product quality[14]
One way to overcome such challenges is by starting potency method development at early development stages. Product characterisation studies help in evaluating product attributes related to potency which are mostly different for each product. During development stages, a qualified potency assay needs to be in place whereas a validated potency assay is needed during the marketing application.
Sponsors shall ensure that potency assays are fully validated and are suitable for their intended purposes. Appropriate justification shall be provided if a single potency assay is performed on the product. Health authorities recommend taking a ‘matrix approach’ at the start of the development due to the complex mechanism of action (MOA) in CGT products. Matrix criteria shall be developed by statistical approach and the results obtained can be considered for setting the final acceptance criteria. In the case of tissue engineered products, health agencies recommend that the potency assay utilise the product in its final form with all its components prepared and formulated together to assess product efficacy for licensure. Sponsors shall ensure that potency assays are part of the stability programme and comparability studies.
Manufacturing process changes and comparability studies
The manufacturing process changes may occur at any stage in the product lifecycle; however, sponsors should ensure that the changes do not negatively impact product safety and quality. Examples of process changes are changes in reagents or process steps and changes to improve an attribute. Risk assessment shall be performed for all manufacturing changes to determine if they have the potential to affect product safety or positively. An analytical comparability study is required for significant changes to the manufacturing process to demonstrate that product quality and safety have not been affected.
The manufacturing process changes may occur at any stage in the product lifecycle…
The level of comparability assessment depends on the stage (early or late phase development) at which the change is proposed, the type of change (minor or major) and patient risk due to the change. Sponsors should investigate conducting prospective side-by-side comparability studies which directly compare the pre-change and post-change product, to eliminate variabilities that can be introduced when using historical data, including differences in analytical methods and changes in the process, other than the change proposed.
Comparability studies are intended to allow the use of clinical data before and after the manufacturing change. If comparability is not established, then the clinical data collected might not be applicable for marketing authorisation application (MAA) and may lead to a requirement for a new clinical study.[4]
Mitigation of challenges
It is important to keep CMC development aligned with clinical development as CMC deficiencies may lead to a clinical hold. It can be particularly challenging to establish quality attributes and measurement of potency, and demonstrate product stability for CGT products. Sponsors shall adopt a stepwise approach based on manufacturing experience to address the issues. Seeking health authorities’ advice in a timely manner e.g. through Pre-IND meetings/scientific advice can provide great support to CMC development.
Analytical aspects are a key area to receive feedback on, especially where a CGT product MOA may not be fully understood. Sponsors must make all possible efforts to ensure that methodologies are sufficient for the current stage of development and that, if the mechanism of action is unknown, surrogate assays can be utilised for further insight. Regulators can help with this by recommending attributes to evaluate.
Another challenge in the diversity of CGT product development is that often-standard practices and processes will not work – for example, some viral vectors are too large or sensitive for terminal sterile filtration. To overcome such issues, one shall determine the best possible alternative using risk-based and scientific justifications for new control strategies. Overall, addressing these challenges with strategic approaches is vital for successful and efficient viral vector manufacturing for GCT in a GMP-compliant environment.
Conclusion
In the evolving field of CGT, breakthrough treatments have emerged for previously incurable diseases. However, the journey from lab to market authorisation is lengthy and financially demanding. Economic barriers, driven by high development and production costs, limit widespread patient access to these therapies. Consequently, some approved CGT products have been withdrawn due to economic challenges and small patient populations. CMC issues play a crucial role in regulatory success, and addressing deficiencies in testing, product stability, raw materials and validation is vital. Seeking timely guidance and close collaboration with health authorities are essential to overcome these challenges and unlock the full potential of CGTs and eventually improve patient outcomes.
References:
[1] El-Kadiry AE-H, Rafei M and Shammaa R. ‘Cell Therapy: Types, Regulation, and Clinical Benefits’, Frontiers in Medicine, 8:756029, 2021.
[2] Lee SK and Lee JH. ‘Cell and gene therapy regulatory, pricing, and reimbursement framework: With a focus on South Korea and the EU’, Frontiers in Public Health, 11:1109873, 24 February 2023.
[3] Sinclair A, Islam S, Jones S. ‘Gene Therapy: An Overview of Approved and Pipeline Technologies’, In: CADTH Issues in Emerging Health Technologies. Ottawa (ON): Canadian Agency for Drugs and Technologies in Health; 2016-2021. 171.
[4] FDA (2023) FDA CBER OTP Town Hall: Cell Therapy Chemistry, Manufacturing and Controls.
[5] American society of Gene + Cell Therapy (ASGCT). Gene, Cell, + RNA Therapy Landscape Report, Q3 2023 Quarterly Data Report.
[6] Alireza Shahryari, Marie Saghaeian Jazi, Saeed Mohammadi et al. ‘Development and Clinical Translation of Approved Gene Therapy Products for Genetic Disorders’. Frontiers in Genetics, 25 September 2019.
[7] Drago D, Foss-Campbell B, Wonnacott K et al. ‘Global regulatory progress in delivering on the promise of gene therapies for unmet medical needs,. Mol Ther Methods Clin Dev.; 21:524-529. PMID: 33997101; PMCID: PMC8099595, 5 April 2015.
[8] Subbu Viswanathan and Marc Puich. ‘CMC obstacles in cell and gene therapy: four solutions to solve six challenges’, Cell & Gene Therapy Insights; 7(5), pp471–488, 2021.
[9] Jote T. Bulcha, Yi Wang, Hong Ma et al. ‘Viral vector platforms within the gene therapy landscape’, Signal Transduction and Targeted Therapy, 2021.
[10] Murali Ramamoorth, Aparna Narvekar. ‘Non-Viral Vectors in Gene Therapy- An Overview’, Journal of Clinical and Diagnostic Research, 9(1), January 2015.
[11] Johannes C.M. van der Loo and J. Fraser Wright. ‘Progress and challenges in viral vector manufacturing’, Human Molecular Genetics, Vol. 25, No. R1 R42–R52, 2016.
[12] Maggie Chen. ‘Overcoming AAV Manufacturing Challenges: Movement Toward Plug-and-Play Solutions’, BioProcess International, 9 February 2023.
[13] John Liddell. ‘Challenges in downstream purification of advanced therapies’, European Pharmaceutical Review, 22 February 2022.
[14] FDA (2011) Guidance for Industry Potency Tests for Cellular and Gene Therapy Products.
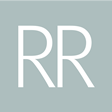